In the first part of this piece, I explain what happens when a receptor is stimulated. I define and present examples of foundational terms, including receptor affinity, full agonist, partial agonist, and antagonist. In the second section, I explain how withdrawal works and how precipitated withdrawal differs from ordinary withdrawal.
I spend some time over on Reddit writing campy r/nosleep horror stories, answering questions about the physiology and pharmacology of opioid addiction, and presenting thinly veiled advertisements for this blog.
One of the phenomena that I consistently read misinformation about is precipitated withdrawal. This isn't surprising, given that understanding receptor dynamics requires detailed knowledge of biochemistry and cellular biology, including how probabilistic quantum effects manifest at this level.
However, this widespread misunderstanding is alarming because - short of death - precipitated withdrawal from opioids is probably the most torturously awful thing that can happen to an addicted body (it's roughly equivalent to having every tissue in your body be hurt in every way that it can be hurt all at once - plus having your neurophysiology plummet into the abyss).
I don't remember much about my experience with precipitated withdrawal, which was triggered by overuse of naloxone (Narcan) by the EMTs and doctors who were treating me for overdose. My memory is probably so limited because I was also high on benzos at the time.
I have, however, heard stories from three friends who have experienced precipitated withdrawal under varying circumstances.*
*Desperate addicts will sometimes induce precipitated withdrawal by taking an opioid antagonist such as naloxone (Narcan) or naltrexone as a last-ditch effort to get clean. I will say more about this later, but if you stop reading now, please know that this never, ever works out, and that it will definitely, certainly, assuredly be one of the three most unpleasant experiences of your life.
To emphasize the importance of avoiding precipitated withdrawal, I'll quote those friends here:
Friend 1: "I thought that I had died and my soul was in hell."
Friend 2: "[Friend 1] is right; it's so dysphoric and terrible that it like takes you to another dimension."
Friend 3: "My mom walked into my bedroom while I was taking a sh*t on the floor..."
Precipitated withdrawal occurs when someone dependent on opioids is put into sudden, severe withdrawal because of a rapid drop in the stimulation of their mu opioid receptors, which can be triggered by ingestion / administration of a partial agonist or an antagonist.
If you just read that definition and thought "I don't know what most or all of that means," then you're in the right place.
I've structured my explanation of precipitated withdrawal around three simple questions:
(1) What is a receptor?
(2) How do receptors work?
(3) How does precipitated withdrawal occur?
First Things First: What Are Receptors?
If you're a Millennial like me, then you might vaguely recall learning about receptors in high school biology.
For today's purposes, a receptor is a protein spanning the cell membrane that binds a molecule (in this case, an opioid drug), which is known as the receptor's ligand. Receptors are highly specific for their ligands, which can either be activating or inhibiting. Binding of activating ligands (known as agonists) leads to changes within the cell that can contribute to a variety of downstream outcomes, such as:
A nerve cell (neuron) releasing a feel-good neurotransmitter like dopamine or an inhibiting neurotransmitter like GABA (gamma-amino butyric acid)
A skeletal muscle cell contracting
An immune cell such as a macrophage engulfing a bacterium and digesting it through oxidative reactions and other processes
Right now, we're concerned with the opioid receptors that bind opioids / opiates like morphine, heroin, oxycodone, and fentanyl. They are found not just on nerve cells (neurons), but also in plentiful numbers in the GI tract.*
*Because there are plentiful mu opioid receptors present on the cell membranes of the epithelial cells of the digestive tract, opioid intoxication causes severe constipation, and, conversely, opioid withdrawal causes nausea / vomiting. One of the most popular and effective over-the-counter antidiarrheal medications, Imodium (loperamide), is an opioid that doesn't cross the blood-brain barrier very efficiently (I've discussed the use of dangerously large quantities of Imodium as a "poor man's methadone" elsewhere).
There are five types of opioid receptors, but the only one that we need to worry about to understand precipitated withdrawal is the mu opioid receptor. It is the "classical" opioid receptor that is responsible for euphoria, analgesia (pain reduction), sedation, and respiratory and heart rate depression.
When opioids are taken regularly for weeks or months, it's changes to the mu opioid receptors that lead to tolerance and addiction.
So, the picture so far is not terribly complicated. On the surface of some cells are the mu opioid receptors, protein structures that bind opioid drugs, which leads to the release of the feel-good neurotransmitter dopamine (as well as other effects in the brain and elsewhere in the body, including the GI tract).

Generic model of receptor action from Principles of Biology. A ligand, in our example an opioid drug, binds to the extracellular domain of a receptor located in the cell membrane, which activates the receptor and triggers an intracellular response. After a time, the ligand dissociates from the receptor, which is then reset and can potentially be activated again. A given cell will have many types of receptors present in its membrane. The cellular response triggered by ligand binding is mediated by second-messenger molecules that are shared by different receptor types, so the response of the cell depends on the net stimulation / inhibition occurring at all of the different receptor types at a given moment.
Okay, we come to a Choose Your Own Adventure juncture. If you're up for a more detailed discussion of mu opioid receptor dynamics, as well as a brief consideration of why we have these receptors in our brains and bodies, read the rest of this section. If you want to nope out, just skip ahead to "How Do Receptors Work?" below.
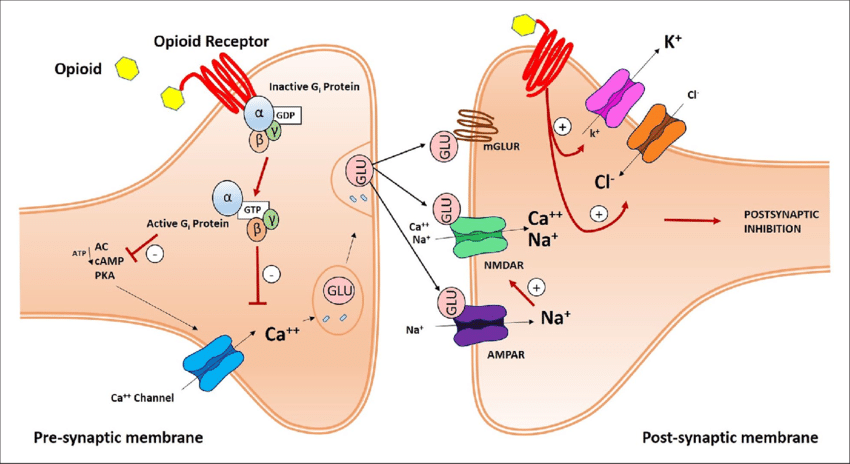
A more realistic and detailed schematic of mu opioid receptor dynamics from a research publication. Unless you've got a solid foundation in cell bio or you're feeling particularly masochistic, don't worry about the details depicted; just try to absorb the overarching concepts.
Here, we can see two nerve cells communicating at a synapse. Opioids bind to the extracellular domain of mu opioid receptors present on the presynaptic nerve cell, which leads to the activation of a molecular complex with three subunits (α, β, and γ) that is linked to the intracellular domain of the receptor, whose activation occurs by phosphorylation of guanosine diphosphate (GDP) to guanosine triphosphate (GTP); the activated complex then effects the intracellular response to ligand binding by inhibiting a calcium channel. The resulting decrease in influx of positively charged calcium ions into the presynaptic cell inhibits the release of glutamate, an excitatory neurotransmitter.
Consequently, less glutamate diffuses across the synapse to the postsynaptic membrane, where it binds to and activates sodium (Na+) and Calcium (Ca++) channels; because activation of these channels inhibits the postsynaptic cell, the decreased activation of these channels that results from opioid receptor activation in the presynaptic cell membrane activates the postsynaptic cell. This postsynaptic cell activation, in turn, can eventually lead to the release of dopamine in the midbrain pleasure centers, for example.
It's a complicated picture incorporating many inverse activation / inhibition effects, but the overall picture is:
Opioid drugs bind to and activate mu opioid receptors in the presynaptic cell membrane --> a three-component molecular complex associated with the intracellular domain of the mu opioid receptors is activated by phosphorylation of GDP to GTP --> Calcium ion channels in the presynaptic cell membrane are inhibited --> Less glutamate is released into the synapse --> Decrease in stimulation of the sodium (Na+) and calcium (Ca++) ion channels in the postsynaptic cell membrane --> Activation of the postsynaptic cell, which produces effects such as release of the euphoria-inducing neurotransmitter dopamine in the midbrain pleasure centers
Molecular and cellular biology are complex and computational. If you've got a good eye for detail, you'll see that there is already a complicating wrinkle in that mu opioid receptors are also found in the post-synaptic cell membrane, where they exert a different, balancing effect mediated by other types of ion channels.
As I tell my students, science hinges on two things: observation and asking the right questions. Some of you might be wondering why the human brain conveniently manufactures proteins to bind opioid drugs, in which case, bravo - you've made your science teachers proud.
In 2024, you've probably heard of endorphins, those feel-good chemicals released by exercise and other, um, activities. Well, "endorphin" is actually a portmanteau of endogenous (meaning made by the body) and morphine. It turns out that we have receptors that bind (exogenous) opioid drugs because our body manufactures similar chemicals that block pain and have feel-good properties.
Maybe now you're wondering why plants make these chemicals (in other words, do plants get high?). The answer is that they make similar chemicals for different reasons (for example, to aid in defense against insects, communication between cells, or because they play a role in cellular metabolism).
All eukaryotes - species such as plants and animals that have nuclei in their cells - manufacture similar families of proteins because much of their genetic code is shared due to common ancestry; evolution always repurposes existing proteins rather than creating novel protein structures, which would be a tremendous waste of metabolic energy.
What we see over the long history of humans and psychoactive plants is that the two have coevolved (evolved together). I touched on this fascinating concept in my discussion of the evolutionary biology of addiction, and I plan to dedicate an entire post to it eventually.
How Do Receptors Work?
A simple way to conceptualize receptor dynamics is that receptors operate like light switches.
Ligands that activate receptors are known as agonists. When an agonist binds to its receptor, the receptor is switched on, and some intracellular response is triggered.
In the case of the mu opioid receptors discussed above, the binding of an opioid drug to the receptor leads to phosphorylation of GDP to GTP, which activates a molecular complex associated with the intracellular domain of the receptor. This, in turn, opens ion channels that lead to other downstream effects (for example, the release of the pleasure-inducing neurotransmitter dopamine within the midbrain pleasure centers).
After an agonist binds to its receptor and the intracellular response is triggered, the ligand eventually dissociates, which leads to the receptor switch being flicked back to the "off" position.
There are also ligands that bind to the receptor and lock it in the off configuration. Such inhibitors are known as antagonists.
For opioid receptors, naloxone (Narcan) and naltrexone are important examples of antagonists. As long as the antagonist is bound to the receptor, no activating intracellular cascade can be initiated.
As is often the case with biology - which a brilliant mentor of mine referred to as the "science of exceptions" - the situation is more complicated than a binary, 0 or 1, on or off response. In addition to full agonists and antagonists, there are also partial agonists.
Because quantum effects come into play at the level of receptor dynamics, we must remember that events are often based on probability rather than certainty. When a partial agonist binds to a receptor, there is a chance that it will be activated, in which case the relevant intracellular chains of events will be triggered.
However, unlike when full agonists bind, when partial agonists bind to their receptors, it is not always the case that the receptor is activated; sometimes there is no response. Depending on the receptor and the ligand, there might be a 0.2 (20%), 0.5 (50%), or 0.9 (90%) chance that the receptor will be activated, for example (see graph below).
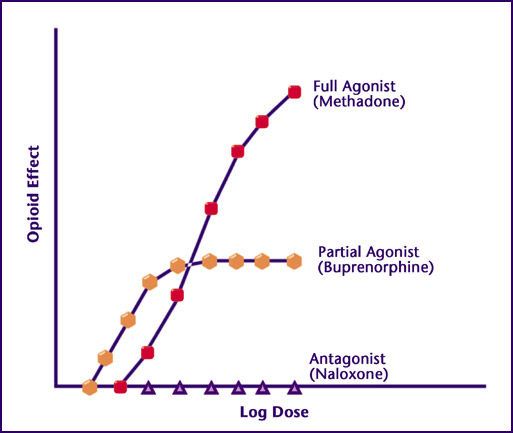
Graph from NAABT.org that shows the level of mu opioid receptor stimulation / opioid effect provided by antagonists, partial agonists, and full agonists. Concentration increases logarithmically from left to right along the horizontal (x-axis); opioid effect, which is proportional to degree of receptor stimulation, increases from bottom to top along the y-axis (vertical). An antagonist locks the receptor in the "off" configuration, so no opioid receptor stimulation occurs while the antagonist is bound (because no agonist can bind to trigger stimulation).
Whereas opioid effect increases proportionally to dose in an indefinite manner with full agonists, we see a ceiling effect with partial agonists, which means that, past a certain point, further increases in dose will not result in more opioid receptor stimulation (the dosage at which this occurs, as well as the corresponding level of opioid receptor stimulation / opioid effect, is unique to each partial agonist and depends on the probability that its binding leads to receptor activation, which can be any value between 0 [0%; antagonist] to 1 [100%; full agonist], not including 0 and 1 themselves).
Another way to summarize these receptor dynamics is through the analogy of a traffic light: A full agonist is the green light, an antagonist is the red light, and a partial agonist is the yellow light in between.
Buprenorphine is one example of a partial agonist whose unique receptor dynamics make it suitable for opioid maintenance therapy.
There is a ceiling effect that manifests with buprenorphine and other partial agonists. At some drug dose / blood concentration, all of the available opioid receptors are bound; the level of mu opioid receptor stimulation is maxed out, and further increasing the blood concentration of buprenorphine will have no effect.
This ceiling effect allows buprenorphine users to stabilize their mu opioid receptor stimulation at a level of receptor stimulation / opioid effect that is less than that provided by full agonists like morphine / heroin, oxycodone, and fentanyl. This level of stimulation is sufficient to prevent most withdrawal symptoms, but not high enough to lead to the full-fledged opioid intoxication that addicts become accustomed to.
Furthermore, because buprenorphine clings very tightly to the mu opioid receptor (see discussion of affinity below), it prevents other full-agonist opioids from binding and causing greater intoxication and / or overdose.
For all of these reasons, buprenorphine is the ideal opioid maintenance treatment. As I have argued elsewhere, it has rendered methadone almost clinically obsolete (more on that here).
Some agonists and antagonists cling to their receptors more tightly than others. We use receptor affinity to describe how tightly a ligand binds to its receptor, which depends on both the rate of association and dissociation from the receptor.
There is a parameter called the dissociation constant, Km, which we use to describe affinity; the lower the Km, the higher the affinity.
Consider, for example, a situation of opioid overdose, in which too many of the opioid receptors are turned on. In this scenario, we need to bind antagonists (naloxone) to as many receptors as possible to make sure that the agonist drug molecules don't bind to and activate the remaining ligand-free receptors.
If we put enough of the antagonist into the patient's blood, we might also be able to push whatever opioid drug the patient has taken off of some of the receptors, thereby decreasing the extent of opioid receptor stimulation and opioid effect, which increases breathing rate, restores consciousness, and so on.
If the opioid that was ingested binds to the mu opioid receptor with very high affinity, however, we might not be able to knock it off the receptors with the antagonists at our disposal.*
*Again, naloxone is the opioid antagonist of choice for overdoses. It is called Narcan and is available in injection and intranasal formulations. I have saved at least two lives by administering Narcan. I have had my own life saved at least three times in this manner (I guess I'm running a deficit, now that I think about it). In my opinion, all U.S. adults should be trained in Narcan use and keep it in their car, purse, desk, or wherever. You might not know that a loved one is an addict until they are overdosing, and in this scenario, Narcan could keep them around. With very few exceptions, administration of Narcan will not hurt the person if it turns out that they are not overdosing on opioids.
One of the reasons that fentanyl overdose kills so many people is that it has a very high binding affinity (see graph below).

Graph from a research paper on mu (μ) opioid receptor binding affinities of various opiates / opioids. You will see many parameters, including Km and Ki, used to quantify affinity. In this example, logarithmic dose increases along the x-axis (horizontal) from left to right, and % receptor binding increases from bottom to top along the y-axis (vertical).
The curves that are left-shifted indicate that these drugs have relatively high receptor affinities; relative to other chemicals with right-shifted curves, they have a higher percentage of bound drug at a lower drug concentration. We can see that sufentanil has one of the highest binding affinities, whereas tramadol, a very weak mu-opioid receptor stimulator that also acts as a serotonin-norepinephrine reuptake inhibitor (SNRI), has the most right-shifted curve, representing the lowest receptor affinity.
Many variables, including molecular composition, GI absorption, and ability to cross the blood-brain barrier, influence how much of a substance is necessary to cause opioid effects. In general, the higher the affinity, the lower the drug dose necessary because the receptors will be bound tightly and efficiently even when the drug concentration isn't very high. Thus, fentanyl and its analogs are active at doses measured in millionths of a gram (micrograms), whereas doses of tramadol require 50 to 100 thousandths of a gram (milligrams), equivalent to 0.05 to 0.1 grams, to exert any appreciable opioid effect. Antagonists, too, have binding affinities.
Arrival of the Cenobites: What Is Withdrawal?
To understand the nature of opioid withdrawal, we've got to get a handle on how the body adapts to chronic opioid intake.
Opioids are powerful depressants with significant effects on nearly every tissue in the body.
For example, ingesting opioids leads to the release of dopamine in the brain, which induces euphoria; decreases breathing rate and heart rate; and slows gastrointestinal mobility (the speed at which food is broken down, absorbed, and moved through the digestive tract).
The body's natural response to regular intake of any exogenous substance is to counteract the effects of that substance. Thus, the body attempts to maintain a state of balance, known as homeostasis, by upregulating substances and triggering physiologic mechanisms that have opposing effects.
In the case of opioids, one of these counteracting substances is a neurotransmitter known as glutamate, which - as I teach my AP Bio students - is sort of like the gas pedal for the central nervous system. Upregulation of glutamate partially opposes the depressant effects of opioids, so - when an addict continues to take opioids for a long period of time - the decreases in heart rate and respiratory rate caused by opioids are less severe.
This phenomenon of decreased physiological response to a drug that occurs with chronic intake is known as tolerance.
It's not just heart rate and respiratory rate that are balanced out with time. The euphoria caused by opioids decreases as well. In fact, one of the nasty little biological quirks involved in addiction is that tolerance to the subjective, psychological effects of the drug, such as euphoria, builds more quickly than tolerance to the objective, physiological effects, such as slowed respiratory and heart rates - putting the user in danger of overdose as he or she chases that euphoric high by taking doses of the drug that cause dangerous depression of the heart and respiratory rates.
With long-term ingestion of a substance, the body can also downregulate and desensitize the receptor system that mediates the drug's effects. For example, it can decrease the number of receptors for the drug present on the cell membrane or render those receptors less responsive to agonists when they bind.
Addiction is the state in which the body has come to depend on a certain level of receptor stimulation, without which it enters withdrawal.
Withdrawal occurs when the addicted person stops ingesting the dose of the substance that their body has come to depend upon, without which it can no longer function normally.
One way to conceptualize withdrawal symptoms is to think of them as the inverses or opposites of the effects caused by ingestion of that drug.
So, because opioids have anticholinergic effects that dry out mucous membranes, during opioid withdrawal, the mucous membranes become overly wet / active - leading to teary eyes (lacrimation), runny nose, and increased saliva production.
Likewise, opioids have a depressant / sedative effect on the central nervous system (CNS), meaning that they decrease its responses to stimuli and dampen its overall speed and level of activity. Because of this, the CNS becomes hyperactive during opioid withdrawal, leading to dilated pupils, oversensitive ears and eyes, racing thoughts, and insomnia.
This depression of the CNS due to opioid ingestion also leads to decreased respiratory and heart rates. Thus, during withdrawal, heart rate and breathing rate both increase (see summary table below).
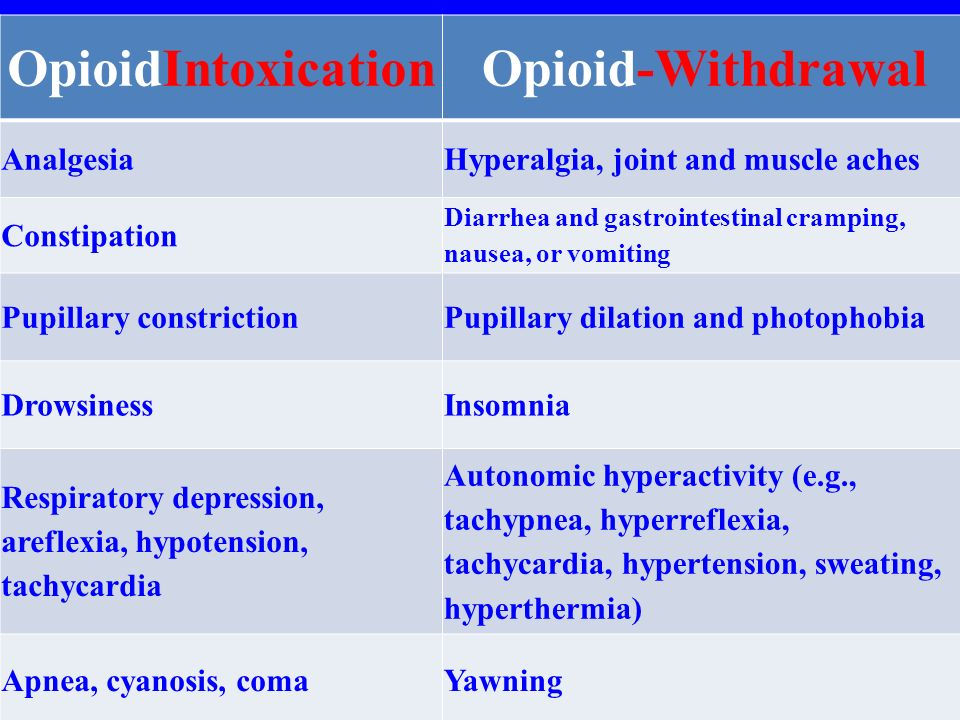
Table of opioid intoxication symptoms versus opioid withdrawal symptoms. Withdrawal symptoms can be thought of as the inverses or opposites of the corresponding symptoms of intoxication. They are caused by a sudden decrease in intake of the drug that the person is addicted to; because that person's body has activated compensatory mechanisms to counterbalance the effects of the drug, his or her physiology is out of balance without it.
Now, opioid withdrawal typically occurs gradually after intake of the substance to which the person is addicted decreases or stops.
The precise timeline for withdrawal onset is correlated with the half-life of the drug of choice - meaning how long the effects of that drug last (a shorter half-life means that they disappear more quickly).
So, for a short-acting opioid such as fentanyl, withdrawal starts within a few hours and peaks within 24 hours. For intermediate-half-life drugs such as morphine and heroin, withdrawal starts after 12 to 14 hours and peaks around 48 to 72 hours. For long-acting opioids such as buprenorphine and methadone, withdrawal begins after 24-48 hours and can take 7 to 10 days or more to peak.
A few hours might not seem like much time for the body to adapt to such a dramatic physiologic change. Indeed, it takes six to eight weeks of abstinence for new receptor creation to begin to reset the affected receptor systems, at which point a return to physiologic baseline ("normal") occurs.
However, even if the body is only given a few hours to self-regulate in response to decreased intake or cessation of a substance, it can activate short-term, balancing responses that significantly mitigate the symptoms of withdrawal.
We arrive at the central issue of this article: Precipitated withdrawal.
Precipitated withdrawal occurs when withdrawal is suddenly initiated by decreasing the level of opioid receptor stimulation via administration of an antagonist, which locks the mu opioid receptors in their "off" configuration.
As discussed above, depending on the receptor affinity of the antagonist relative to the receptor affinity of the opioid drug that has been ingested, the antagonist might even knock some of the opioid drug off of the receptors that its bound to and activating.
This results in a sudden, full-intensity withdrawal syndrome that the body has had no time to adapt to, leading to physiologic shock that further amplifies the withdrawal symptoms. Precipitated withdrawal is a waking nightmare; it is one of the worst things that can happen to an addicted body.
If too much naloxone (Narcan) is administered during an overdose, precipitated withdrawal is triggered.*
*I have experienced this myself; zero stars on Yelp - absolutely do not recommend. There are some kinds of pain that take a permanent chunk out of your soul.
Crucially, precipitated withdrawal can also occur when a partial agonist is given to someone dependent on a high enough dose of a full agonist.
If you refer back to the first image in the "How Do Receptors Work?" section, which shows opioid effect on the y-axis versus logarithmic dose on the x-axis, you see that - beyond a certain dose, at which the ceiling effect of the partial agonist is achieved - no more opioid effect is generated by further increases in dose.
Now, if someone is dependent on a dose of a full agonist that is below or equal to the equivalent partial agonist dose at which the ceiling effect of the partial agonist comes into play, everything is kosher - when the ceiling dose of the partial agonist is administered, there will be enough opioid receptor stimulation to keep the person's body happy.
Beyond that dose, however, precipitated withdrawal occurs because the partial agonist cannot provide enough opioid receptor stimulation to keep the body at the level that it is used to (and thus out of withdrawal).
The severity of the precipitated withdrawal symptoms will be proportional to the gap between the two curves (in other words, the difference between the level of opioid receptor stimulation / opioid effect that the addicted person's body is used to versus the ceiling level of receptor stimulation / opioid effect that the partial agonist is able to provide).
This comes into play with the two drugs used for opioid maintenance therapy in the U.S., which are methadone and buprenorphine.
Methadone is a full agonist, whereas buprenorphine is a partial agonist. The ceiling effect of buprenorphine, which most patients reach at a daily dose of 8 to 16 milligrams of sublingual buprenorphine, is equivalent to the opioid stimulation provided by about 25 to 40 milligrams of (oral) methadone.
For this reason, most clinics in the U.S. traditionally recommended that methadone patients decrease their dose to 25 to 40 milligrams before switching to buprenorphine. However, there are innovative, rapid transition protocols that do not require this difficult-to-achieve reduction, which I discuss in a forthcoming post.
There are many online opioid dosage converters, which are handy if you're switching from one opioid to another and you want to ensure maintenance of the same level of opioid receptor stimulation so that your body experiences neither intoxication nor withdrawal.
This one from the Oregon Pain Guidance site uses morphine equivalents to convert between substances. Keep in mind that there is significant variability in these converters; part of this is because some of them focus on pain reduction, whereas others use respiratory depression or other parameters to gauge equivalence.
Pay attention to route of administration (how the drug gets into your body), as well. This is particularly important for drugs such as morphine, which require much larger oral dosages compared to injected or sublingual doses because they are subject to extensive first-pass metabolism by the liver.
Whenever you switch from one opioid to another, you will benefit from a phenomenon known as incomplete cross-tolerance, which means that you will likely need 5% to 15% less of the new opioid than these equivalent dosage calculators predict (unless you are using one of the calculators that builds incomplete cross-tolerance into its model).
Pain management protocols constructively use this effect by periodically rotating patients from one opioid agent to another, which slows the development of tolerance and the need for dose increases.
Congratulations! (No Sarcasm Intended, For Once)
If you've made it this far and understood 50 to 75 percent of what you've read, then you're well on your way to a solid understanding of opioid receptor dynamics, which is crucial to making decisions about pain management and opioid addiction treatment as well as interpreting the exciting research that is being undertaken at the moment.
Speaking of that research, my editorial calendar - which is currently crowded by 104 planned pieces, which I will hopefully churn out more quickly now that school is out for the summer - includes a piece on the simultaneous use of agonists and antagonists.
While logic seems to dictate that either the agonist effects will outweigh the antagonist effects or vice-versa, in reality, the receptor dynamics are much more complex and interesting. In fact, it turns out that such an agonist / antagonist pairing might allow effective treatment of pain without the buildup of tolerance even when the opioid is used for extended periods.
I also plan to write about the delta and kappa opioid receptors, which have completely different effects compared to the mu opioid receptors, some of which counteract the mu opioid receptor's effects. For this and other reasons, these receptors are promising therapeutic targets.
In addition, the very next science-y post that I have planned discusses novel, rapid protocols for transition from methadone to buprenorphine.
Community Updates
I'll be happy to answer any questions about specific clinical situations below (keeping in mind that, although I finished my first year of medical school, I'm far from a doctor, and you should use any feedback that I provide to inform your discussions with the appropriate clinical professionals).
Please follow me on Instagram. Although there's not much activity over there yet, at the moment, it's the easiest way to communicate aside from the Contact form here.
We're currently nearing 90 days since the blog went online, and the number of views that I'm getting is beyond what I expected for the first 9-12 months. This is wonderful, of course, but it also means that I've had to adjust my plan for building out this Concrete Confessional project. At the moment, I'm postponing the launch of our Entropy, Inc., Discord server.
Once again, thank you for reading! Your interest and support mean more to me than you could know.
I'm still in the middle of an Arduous March of a methadone taper, which I hope to finish by the end of the summer, and having this project to occupy myself and assist others is saving me.
Comments